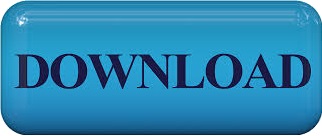
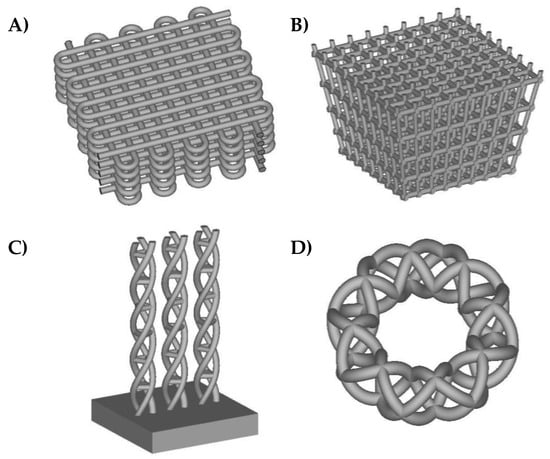
Recently, the concept of 4D materials has emerged, where a 3D-printed material displays behavior such as shape memory, swelling, or controlled degradation in a 4th dimension, typically time 5, 22, 30, 31. An alternative synthetic materials platform that can balance minimally invasive behavior with nutrient/waste diffusion and support tissue regrowth as they degrade in vivo to nontoxic byproducts without succumbing to the limitations of current tissue engineering therapies is needed. The primary 3D printing material focus has remained on acrylate- and epoxide-containing polymers, which have low toxicity thresholds, while the primary degradable biomaterial focus has been directed towards poly( l-lactic acid) (PLLA), which is limited by its poor processability in photopolymerizations and its acidic degradation products 25, 26, 27, 28, 29. However, a significant current limitation in the field is the lack of suitable materials that can be processed efficiently by additive manufacturing techniques, particularly in the layer-integrating photopolymerization methods, coupled with long-term biocompatibility in vivo 5, 17, 22, 23, 24, 25, 26, 27. 3D printing has emerged as one of the most promising methods to overcome these processing limitations as a consequence of the reproducible, interconnected pore features with micron-scale resolution that can be manufactured in precise designs. However, current porous materials leveraged for these applications are limited by their pore morphological distribution, in addition to other issues such as post-processing requirements prior to use 5, 6, 7, 9, 10, 11, 12, 13, 14, 15, 16, 17, 18, 19, 20, 21. This concept has been successfully demonstrated in applications such as siloxane maxillofacial implants, polyurethane cardiovascular occlusive devices, and collagen-derived adipose tissue scaffolds 5, 6, 7, 8, 9, 10, 11. Porous materials in particular offer tissues the mechanical support that is necessary for them to rapidly infiltrate a space. Tissue scaffold design has the potential to revolutionize patient care, with supportive materials being essential to resolve wounds that are otherwise untreatable. An example of this is in soft tissue repair, such as following breast cancer treatment, a mentally and physically debilitating cancer affecting millions of patients globally 1, 2, 3, 4. Despite these evolutionary materials templates, tissue engineering is currently limited by an urgent, unmet need for improved restorative and healing techniques to address tissue defects. Nature has created tissue designs that are ideally suited for their purpose: bone possesses high strength with enough flexibility to not be brittle arteries are elastomeric without being flimsy and adipose tissue is soft and yielding while being durable. Notably, reduced collagen capsule thickness indicates that these scaffolds are highly promising for adipose tissue engineering and repair. In vivo, adipocyte lobules were found to infiltrate the surface-eroding scaffold within 2 months, and neovascularization was observed over the same time. The 4D nature of the printed scaffolds is manifested in their shape memory properties, which allows them to fill model soft tissue voids without deforming the surrounding material. Herein, soft, elastomeric aliphatic polycarbonate-based materials were designed to undergo photopolymerization into supportive soft tissue engineering scaffolds. However, there is a need for improved minimally invasive, void-filling materials to provide mechanical support, biocompatibility, and surface erosion characteristics to ensure consistent tissue support during the healing process. 3D printing has emerged as one of the most promising tools to overcome the processing and morphological limitations of traditional tissue engineering scaffold design.
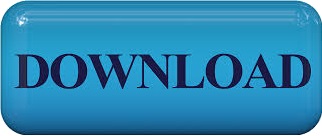